Meet the Women and Men Winning the Fight Against Cancer
Meet the men and women working to beat cancer.
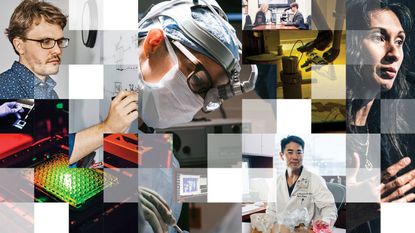
The men and women who are trying to bring down cancer are starting to join forces rather than work alone. Together, they are winning a few of the battles against the world's fiercest disease. For this unprecedented special report, we visited elite cancer research centers around the country to find out where we are in the war.
I. Precision Medicine: What Is Cancer, Really?
When you visit St. Jude Children's Research Hospital in Memphis, Tennessee, you expect to feel devastated. It starts in the waiting room. Oh, here we go with the little red wagons, you think, observing the cattle herd of them rounded up by the entrance to the Patient Care Center. Oh, here we go with the crayon drawings of needles. The itch begins at the back of your throat, and you start blinking very fast and mentally researching how much money you could donate without starving. Near a row of arcade games, a preteen curls his face into his mother's shoulder while she strokes his head. Oh, here we go.
But the more time you spend at St. Jude, the more that feeling is replaced with wonder. In a cruel world you've found a free hospital for children, started by a Hollywood entertainer as a shrine to the patron saint of lost causes. There is no other place like this. Corporations that have nothing to do with cancer—nothing to do with medicine, even—have donated vast sums of money just to be a part of it. There's a Chili's Care Center. The cafeteria is named for Kay Jewelers.
Scott Newman's office is in the Brooks Brothers Computational Biology Center, where a team of researchers is applying computer science and mathematics to the question of why cancer happens to children. Like many computer people, Newman is very smart and a little quiet and doesn't always exactly meet your eyes when he speaks to you. He works on St. Jude's Genomes for Kids project, which invites newly diagnosed patients to have both their healthy and tumor cells genetically sequenced so researchers can poke around.
"Have you seen a circle plot before?" Newman asks, pulling out a diagram of the genes in a child's cancer. "If I got a tattoo, it would be one of these." Around the outside of the circle plot is something that looks like a colorful bar code. Inside, a series of city skylines. Through the center are colored arcs like those nail-and-string art projects students make in high school geometry class. The diagram represents everything that has gone wrong within a child's cells to cause cancer. It's beautiful.
A Genetic Disaster: This circular visualization shows real gene mutations found in 3,000 pediatric cancers at St. Jude Children\'s Research Hospital. Genes with sequence mutations are labeled in blue; those with structural variations are in red; and those
"These are the genes in this particular tumor that have been hit," Newman says in a Yorkshire accent that emphasizes the t at the end of the word hit in a quietly violent way. "And that's just one type of thing that's going on. Chromosomes get gained or lost in cancer. This one has gained that one, that one, that one, that one," he taps the page over and over. "And then there are structural rearrangements where little bits of genome get switched around." He points to the arcs sweeping across the page. "There are no clearly defined rules."
It's not like you don't have cancer and then one day you just do. Cancer—or, really, cancers, because cancer is not a single disease—happens when glitches in genes cause cells to grow out of control until they overtake the body, like a kudzu plant. Genes develop glitches all the time: There are roughly twenty thousand genes in the human body, any of which can get misspelled or chopped up. Bits can be inserted or deleted. Whole copies of genes can appear and disappear, or combine to form mutants. The circle plot Newman has shown me is not even the worst the body can do. He whips out another one, a snarl of lines and blocks and colors. This one would not make a good tattoo.
"As a tumor becomes cancerous and grows, it can accumulate many thousands of genetic mutations. When we do whole genome sequencing, we see all of them," Newman says. To whittle down the complexity, he applies algorithms that pop out gene mutations most likely to be cancer-related, based on a database of all the mutations researchers have already found. Then, a genome analyst manually determines whether each specific change the algorithm found seems likely to cause problems. Finally, the department brings its list of potentially important changes to a committee of St. Jude's top scientists to discuss and assign a triage score. The mutations that seem most likely to be important get investigated first.
Stay In The Know
Marie Claire email subscribers get intel on fashion and beauty trends, hot-off-the-press celebrity news, and more. Sign up here.
It took thirteen years and cost $2.7 billion to sequence the first genome, which was completed in 2003. Today, it costs $1,000 and takes less than a week. Over the last two decades, as researchers like Newman have uncovered more and more of the individual genetic malfunctions that cause cancer, teams of researchers have begun to tinker with those mutations, trying to reverse the chaos they cause. (The first big success in precision medicine was Gleevec, a drug that treats leukemias that are positive for a common structural rearrangement called the Philadelphia chromosome. Its launch in 2001 was revolutionary.) Today, there are eleven genes that can be targeted with hyperspecific cancer therapies, and at least thirty more being studied. At Memorial Sloan Kettering Cancer Center in New York City, 30 to 40 percent of incoming patients now qualify for precision medicine studies.
Charles Mullighan,a tall, serious Australian who also works at St. Jude, is perhaps the ideal person to illustrate how difficult it will be to cure cancer using precision medicine. After patients' cancer cells are sequenced, and the wonky mutations identified, Mullighan's lab replicates those mutations in mice, then calls St. Jude's chemical library to track down molecules—some of them approved medicines from all over the world, others compounds that can illuminate the biology of tumors—to see if any might help.
New York: Britta Weigelt and Jorge Reis-Filho use police forensics techniques to repair old tumor samples at Memorial Sloan Kettering so the samples can be genetically profiled.
If Mullighan is lucky, one of the compounds he finds will benefit the mice, and he'll have the opportunity to test it in humans. Then he'll hope there are no unexpected side effects, and that the cancer won't develop resistance, which it often does when you futz with genetics. There are about twenty subtypes of the leukemia Mullighan studies, and that leukemia is one of a hundred different subtypes of cancer. This is the kind of precision required in precision cancer treatment—even if Mullighan succeeds in identifying a treatment that works as well as Gleevec, with the help of an entire, well-funded hospital, it still will work for only a tiny proportion of patients.
Cancer is not an ordinary disease. Cancer is the disease—a phenomenon that contains the whole of genetics and biology and human life in a single cell. It will take an army of researchers to defeat it.
Luckily, we've got one.
Interlude
"I used to do this job out in L.A.," says the attendant at the Hertz counter at Houston's George Bush Intercontinental Airport. "There, everyone is going on vacation. They're going to the beaach or Disneyland or Hollywood or wherever.
"Because of MD Anderson, I see more cancer patients here. They're so skinny. When they come through this counter, they're leaning on someone's arm. They can't drive themselves. You think, there is no way this person will survive. And then they're back in three weeks, and in six months, and a year. I'm sure I miss some, who don't come through anymore because they've died. But the rest? They come back."
II. Checkpoint Inhibitor Therapy: You Have the Power Within You!
On a bookshelf in Jim Allison's office at MD Anderson Cancer Center in Houston (and on the floor surrounding it) are so many awards that some still sit in the boxes they came in. The Lasker-DeBakey Clinical Medical Research Award looks like the Winged Victory statue in the Louvre. The Breakthrough Prize in Life Sciences, whose benefactors include Sergey Brin, Anne Wojcicki, and Mark Zuckerberg, came with $3 million.
"I gotta tidy that up sometime," Allison says.
Allison has just returned to the office from back surgery that fused his L3, L4, and L5 vertebrae, which has slightly diminished his Texas rambunctiousness. Even on painkillers, though, he can explain the work that many of his contemporaries believe will earn him the Nobel Prize: He figured out how to turn the immune system against tumors.
"One day, the miracles won't be miracles at all. They'll just be what happens."
Allison is a basic scientist. He has a Ph.D., rather than an M.D., and works primarily with cells and molecules rather than patients. When T-cells, the most powerful "killer cells" in the immune system, became better understood in the late 1960s, Allison became fascinated with them. He wanted to know how it was possible that a cell roaming around your body knew to kill infected cells but not healthy ones. In the mid-1990s, both Allison's lab and the lab of Jeffrey Bluestone at the University of Chicago noticed that a molecule called CTLA-4 acted as a brake on T-cells, preventing them from wildly attacking the body's own cells, as they do in autoimmune diseases.
Allison's mother died of lymphoma when he was a child and he has since lost two uncles and a brother to the disease. "Every time I found something new about how the immune system works, I would think, I wonder how this works on cancer?" he says. When the scientific world discovered that CTLA-4 was a brake, Allison alone wondered if it might be important in cancer treatment. He launched an experiment to see if blocking CTLA-4 would allow the immune system to attack cancer tumors in mice. Not only did the mice's tumors disappear, the mice were thereafter immune to cancer of the same type.
Ipilimumab ("ipi" for short) was the name a small drug company called Medarex gave the compound it created to shut off CTLA-4 in humans. Early trials of the drug, designed just to show whether ipi was safe, succeeded so wildly that Bristol Myers Squibb bought Medarex for $2.4 billion. Ipilimumab (now marketed as Yervoy) became the first "checkpoint inhibitor": It blocks one of the brakes, or checkpoints, the immune system has in place to prevent it from attacking healthy cells. Without the brakes the immune system can suddenly, incredibly, recognize cancer as the enemy.
"You see the picture of that woman over there?" Allison points over at his desk. Past his lumbar-support chair, the desk is covered in papers and awards and knickknacks and frames, including one containing a black card with the words "Never never never give up" printed on it. Finally, the photo reveals itself, on a little piece of blue card stock.
"That's the first patient I met," Allison says. "She was about twenty-four years old. She had metastatic melanoma. It was in her brain, her lungs, her liver. She had failed everything. She had just graduated from college, just gotten married. They gave her a month."
The woman, Sharon Belvin, enrolled in a phase-two trial of ipilimumab at Memorial Sloan Kettering, where Allison worked at the time. Today, Belvin is thirty-five, cancer- free, and the mother of two children. When Allison won the Lasker prize, in 2015, the committee flew Belvin to New York City with her husband and her parents to see him receive it. "She picked me up and started squeezing me," Allison says. "I walked back to my lab and thought, Wow, I cure mice of tumors and all they do is bite me." He adds, dryly, "Of course, we gave them the tumors in the first place."
After ipi, Allison could have taken a break and waited for his Nobel, driving his Porsche Boxster with the license plate CTLA-4 around Houston and playing the occasional harmonica gig. (Allison, who grew up in rural Texas, has played since he was a teenager and once performed "Blue Eyes Crying in the Rain" onstage with Willie Nelson.) Instead, his focus has become one of two serious problems with immunotherapy: It only works for some people.
So far, the beneficiaries of immune checkpoint therapy appear to be those with cancer that develops after repeated genetic mutations—metastatic melanoma, non-small-cell lung cancer, and bladder cancer, for example. These are cancers that often result from bad habits like smoking and sun exposure. But even within these types of cancer, immune checkpoint therapies improve long-term survival in only about 20 to 25 percent of patients. In the rest the treatment fails, and researchers have no idea why.
Lately, Allison considers immune checkpoint therapy a "platform"—a menu of treatments that can be amended and combined to increase the percentage of people for whom it works. A newer drug called Keytruda that acts on a different immune checkpoint, PD-1, knocked former president Jimmy Carter's metastatic melanoma into remission in 2015. Recent trials that blocked both PD-1 and CTLA-4 in combination improved long-term survival in 60 percent of melanoma patients. Now, doctors are combining checkpoint therapies with precision cancer drugs, or with radiation, or with chemotherapy. Allison refers to this as "one from column A, and one from column B."
The thing about checkpoint inhibitor therapy that is so exciting—despite the circumscribed group of patients for whom it works, and despite sometimes mortal side effects from the immune system going buck-wild once the brakes come off—is the length of time it can potentially give people. Before therapies that exploited the immune system, response rates were measured in a few extra months of life. Checkpoint inhibitor therapy helps extremely sick people live for years. So what if it doesn't work for everyone? Every cancer patient you can add to the success pile is essentially cured.
Jennifer Wargo and team remove lymph nodes from a melanoma patient.
Jennifer Wargo is another researcher at MD Anderson who is trying to predict who will respond to checkpoint inhibitor therapy and who will not. Originally a nurse, Wargo got so interested in biology that she went back to school for a bachelor's degree, then a medical degree, and then a surgical residency at Harvard. It was during her first faculty position, also at Harvard, around 2008, that she started to wonder how the microbiome—the bacteria that live in the human body, of which there are roughly 40 trillion in the average 155-pound man—might affect cancer treatment. Wargo was investigating the bacteria that lived near the site of pancreatic cancer—in and around the tumor. Could you target those bacteria with drugs and make the cancer recede more quickly?
In the early 2010s, research about the microbiome in the human gut—the bacteria in humans' stomachs and intestines that appear to affect immune function, gene expression, and mood, among other things—gained traction in journals. Before long, two separate researchers had shown that you could change a mouse's response to immune checkpoint inhibitor therapy by giving him certain kinds of bacteria. Wargo added the microbiome to her slate of experiments. Along with her team, she collected gut microbiome samples from more than three hundred cancer patients who then went on to receive checkpoint inhibitors as treatment. The results were, Wargo says, "night and day." People who had a higher diversity of gut bacteria had a stronger response to checkpoint inhibitor therapy.
Now, Wargo is transplanting stool samples from patients into germ-free mice with melanoma, to see if she can predict whether the mice will mimic the treatment responses of the people whose bacteria they received. "Can we change the gut microbiome to enhance responses to therapy . . . or even prevent cancer altogether?" she says. "Ah god, that would be the holy grail, wouldn't it?" she whispers, as if not to invite bad luck. "It's gonna take a lot of work to get there, but I think the answer is gonna be yes."
Immunotherapies do have one other problem worth worrying about, one that underlies the most frustrating experience of having cancer. When a patient is diagnosed, the first therapy is still one of the standards: surgery, radiation, or chemotherapy. Cut, burn, or poison, as the doctors say. Doctors can't use promising immunotherapies as first-line treatments yet because immunotherapies are still dangerous: No one knows what will happen long-term if you shut off the immune system's brakes. Does a patient survive cancer just to develop another terrible disease, like amyotrophic lateral sclerosis (ALS), in fifteen years?
Interlude
"Just to play devil's advocate," says a woman at a margarita bar and restaurant in Santa Fe, New Mexico. "Don't you think the cure exists somewhere already and the medical industrial complex is hiding it? People stand to lose billions of dollars. Don't you think they want to keep that money?"
I have been talking to this woman for twenty minutes. She is familiar with cancer. She works with natural cures, is a big fan of neuroscience, and knows some of the prominent names in medical research. I tell her that the conspiracy theory she is referencing—that the government or pharmaceutical industry is hiding the cure for cancer—can't be true. Of course it's hard to believe that Richard Nixon initiated the war on cancer in 1971 and the disease still kills 595,690 people a year. And that the most brilliant minds of our time have turned HIV into a chronic disease but cancer continues relatively unchecked. And yet I've talked to thirty-five researchers and policymakers and visited seven cancer centers and I haven't seen a shred of evidence that doctors who treat very sick people—and whose job it is, sometimes, to tell people that they will die—aren't trying with their very souls to succeed at their jobs.
"It's just that it's hard," I say.
The woman huffs. Someone more interesting is sitting on the other side of her. And that's the end of that.
The Magic Isotope
The appeal of actinium-225 as a cancer drug is in the alpha particles it releases. Alpha particles have such low tissue penetration that they can't even pass through a sheet of paper, so in theory they could kill cancer cells without causing many side effects. If a drug company could attach a targeting mechanism to the actinium-225, the isotope would attack cancer cells with alpha particles as it decays into francium-221, astatine-217, and bismuth-213. The fourth decay can occur by two different routes, with each path releasing one alpha particle and one beta particle before reaching lead-209. The small amount of stable bismuth-209 that remains at the end is excreted in the urine.
III. CAR-T Cells: Tiny Machines
On a shelf in Crystal Mackall's office at Stanford University in Palo Alto, California, catty-corner to a window that looks out on a lovely California scrub scape, is a teddy bear that once belonged to a boy named Sam.* Sam, who Mackall treated at the National Cancer Institute more than ten years ago, had Ewing's sarcoma, a rare cancer that usually affects children and grows in or around bones.
Mackall is a pediatric oncologist with a dark blond bob and a wry, take-no-prisoners sense of humor. She has worked on cancer since the 1980s, so she has met a lot of very, very sick children. The way Mackall tells the story of Sam, like she's taking a shot of foul-tasting medicine, you can see the distance she's had to put between her emotions and her work. "We lost Sam. He was ten," she says. "We gave him immunotherapy and it didn't work."
With that, Mackall moves on to the story of a girl named Lisa, who is pictured in a photo not far from the bear. Lisa had the same illness as Sam around the same time, but her therapy did work. Lisa's story lasts more than a minute, with Mackall practically cheering at the end. "So she remained fertile and that's her little boy!" she yells, gesturing toward Lisa's photo. Mackall smiles the pained, confused smile of someone who has inexplicably survived a car crash. "You have your ups and your downs," she says.
Houston: A neurofeedback session at MD Anderson, designed to reduce nerve pain caused by chemotherapy.
Overall, children's cancer has been one of the great success stories in cancer treatment. In the 1970s, dramatic advances in chemotherapy put most patients with certain types of leukemia (particularly acute lymphoblastic leukemia in B-cells, otherwise known as B-ALL) into remission. Today, 84 percent of children who get ALL can be cured. But then treatment stalled. "We have made steady progress, by all accounts," says Mackall. "But it's been largely incremental. And there've been these plateaus that have just driven us crazy."
In those unfortunate few children who relapsed or didn't respond to the chemo, or who got a different variety of cancer, like Ewing's sarcoma, there were few treatments left to try. Mackall's patients came to her after having had surgery and then chemotherapy once, twice, three times. "You can just see, they're beat up. They're making it, but all they do is get their treatments," she says. "They didn't have enough energy to do anything else." And then, if they lasted long enough, they got into a trial.
There are several ways to turn the immune system against cancer. Checkpoint inhibitor therapy is one of them. But it doesn't work in all patients, especially children, whose cancers generally do not have the vast numbers of mutations needed to attract the attention of a newly brake-free immune system. For a long, dark time, immunotherapists would try other sorts of techniques to get the immune system to respond in these patients, and the patients would die anyway, like Sam did. The treatments were toxic or they damaged the brain or they just didn't work. The doctors would recommend hospice. Hospice. Hospice. Hospice.
And then all the research began to pay off. In August 2010, a retired correctional officer named Bill Ludwig walked into the Hospital of the University of Pennsylvania to try a new therapy developed by a researcher named Carl June. Ludwig had chronic lymphocytic leukemia (CLL), another cancer that affects B-cells. Multiple rounds of chemotherapy had failed to cure it, and he didn't qualify for a bone marrow transplant. June's idea, which was so risky that the National Institutes of Health had turned down several grant applications to fund it, was the only option Ludwig had. June had only enough money to try it in three patients. Ludwig went first.
To understand how June's therapy works, consider the T-cells that Jim Allison found fascinating. They're cells that kill other cells, but they don't kill you because they have a built-in targeting mechanism. Each person has millions of T-cells, and each one of those T-cells matches a single virus, like a lock and a key. If a virus enters the body, its own personal T-cell key will find and destroy it, then copy and copy and copy itself until the virus succumbs. "I liken it to a bloodhound," says Mackall. "What the marker says to the T-cell is: Anything that has this thing on it, kill it."
Previously, researchers had created a fake key called a chimeric antigen receptor, or CAR, that matched a particular lock, CD19, on B-cells, which is where Ludwig's leukemia was. During the trial, Ludwig's doctors removed as many of Ludwig's T-cells as they could, and June's team inserted the CAR using a modified form of HIV, which can edit genes. Then they returned the T-cells to Ludwig.
Ten days later, Ludwig started to have chills and fever, like he had the flu. He was so ill that doctors moved him to the intensive care unit. But then, less than a month later, he was in remission. The T-cells had located and demolished the cancer, the same way they would a virus.
New York: Dan Heller, a researcher at Memorial Sloan Kettering Cancer Center, whose lab built this custom supercontinuum laser infrared plate reader, is developing an implantable cancer sensor made of nanocarbon. When Heller implanted a prototype sensor i
When case studies of the first three patients were published in scientific journals, mainstream media went crazy: "Cancer treated with HIV!" they shouted. But it was a later study that showed that the furor was warranted: When the Penn team partnered with the Children's Hospital of Pennsylvania to try CAR-T cell therapy against B-ALL in children, the cancer disappeared in twenty-four out of twenty-seven patients.
Novartis was the drug company that partnered with the University of Pennsylvania to turn June's treatment into a drug for the general public, and the company submitted results of all three required levels of tests to the Food and Drug Administration early this year. If the FDA approves the drug, any child who has B-ALL and has failed her first therapy can have her white blood cells removed, frozen, and shipped to Novartis's processing facility in Morris Plains, New Jersey, where molecular engineers will insert the new "key" and send the T-cells back. The patient gets a one-time infusion, and there's an 83 percent chance she will be cured.
"We also do a second measure of remissions where we look to see if there's any measurable disease at all," says David Lebwohl, Novartis's global program head for CAR-T treatments. "A more sensitive test than just looking in the blood. And that was also negative for 83 percent of the patients."
An 83 percent cure rate in children who would otherwise die is a monumental achievement. If there is a moment where a culture hits on an idea that can cure a disease—vaccines, for example, or penicillin—we are in it. It is difficult to overstate this: Humans have been trying to create a cell therapy for cancer patients for generations. "People said: That can't be done, You can't make them from cancer patients, You can't make them, You can't get them, It's too complicated," says Crystal Mackall. "But it's happening." Though Novartis couldn't confirm an official release date, Mackall suspects the drug will become widely available this year.
Cancer being cancer, of course, there are limitations: Until it clears further FDA hurdles, Novartis's drug will be available only for children with B-ALL and not for any of the dozens of other types of cancers that affect children and adults. In solid tumors, the CAR-T cells aren't strong enough to kill the whole thing, or they die before they finish the job. Worse, once attacked, some leukemia cells will remove their CD19 proteins and go back into hiding. "The thing about cancer is, it's quite a foe," Mackall says. "The minute you think you've got the one thing for it, it'll outsmart you."
Slowly, though, the successes are mounting. At City of Hope National Medical Center just outside Los Angeles, Behnam Badie, an Iranian-born brain surgeon who has the kind of bedside manner you'd dream of if you ever required a brain surgeon, is developing a surgical device that can continuously infuse CAR-T cells into the brain tumors of cancer patients while he operates. For a while, he was working with the California Institute of Technology to build a magnetic helmet that could move the cells to the correct places, but the project ran out of money.
Meanwhile, Crystal Mackall is working on a backup target for the CAR-T cells, CD22, in case a child's cancer resists the ones targeted to CD19. She is also trying to make the cells live longer. Working with similar but slightly different engineered cells, she has managed to get her therapy to stay alive and working for up to two years in patients with solid sarcomas. One of her patients has since gotten married and bought a farm. Another went on a volunteer trip to Africa.
Mackall likens genetically engineered cells to rudimentary machines. Over the next decade, she says, scientists will refine them until they can control where they go and what they do and when. "We're going to be in a situation," she says, "where a doctor can tell a patient to take pills to activate his cells one week and then rest them the next." In fact, a biotech company based in San Diego called BioAtla has already developed conditionally active markers that could tell a T-cell to kill or not kill based on where it is in the body.
Palo Alto: Stanford\'s Crystal Mackall is developing cancer-fighting cell machines.
Eventually, programmable cell machines could fight autoimmune diseases, or arthritis. They could be used to rebuild collagen in athletes' knees. But, because such powerful new technology requires a ton of risk to attempt, none of this would have been developed without an adversary as vile as cancer to require it. "We treated forty-nine kids at the National Cancer Institute with refractory leukemia. Every single one of those kids had exhausted every other therapy available. If it weren't for the CAR-T cells, they were gonna die," Mackall says. Sixty percent of those children went into remission, and a sizable fraction of those appear to be cured. "You're able to take the chance only in that situation, when people don't have other options."
People will die waiting for CAR-T therapy to really, truly happen. In the United States, doctors aren't permitted to experiment on patients who have other options, and it will take a long time for CAR-T to prove itself better than the treatments already available. But someone has to choose to take the first walk down the path to the future. In a final act that is equal parts self-preservation and sacrifice, that person is usually a cancer patient. And soon, more of them will be able to make the decision for themselves.
Interlude
"What're ya down here for?" asks an older gentleman at the bar of a tourist barbecue joint near my hotel in Memphis. I'm halfway through a plate of pickles and dry-rubbed ribs. I explain that I've spent all day at St. Jude.
"God bless you," he says. "I couldn't do it." The man is from Texas—he works in shipping or packing or something or other.
The bartender, a bubbly twenty-three-year-old, offers the gentleman another beer. "You know, I was treated at St. Jude. Diagnosed at ten. Cured at thirteen," he says, beaming.
"Was it awful?" I ask. "Getting cancer as a kid?"
"Naw, I loved going to St. Jude. I remember I looked forward to school being over so I could go over to the hospital and get chemo. Your doctors are so happy to see you."
The bartender is studying to be a truck driver so he can visit California. He's not sure if he'll settle down there, but it seems nice.
The man from Texas looks at the bartender hard for a good minute, says, "You're a lucky man, son."
IV. Postmodern Radiation: Any Other Ideas?
Los Alamos: Rain falls on the National Security Sciences Building.
To get to the Los Alamos National Laboratory in New Mexico, you drive from Santa Fe through peach-parfait mesas and off into the sunset. Even on the public roads, there are checkpoints where security officers will ask to see your driver's license. The deeper you go, the more intense the screening gets, until finally you end up in a place employees just call "behind the fence."
After the public roads but before "behind the fence," are the hot cells: four-foot by three-and-a-half-foot boxes where employees use robot hands controlled by joysticks to process non-weapons-grade isotopes. The isotopes are made on another mesa, by a linear particle accelerator that shoots rare metals with proton beams.
Just outside the hot cells, Eva Birnbaum, the isotope production facility's program manager, asks me if I know what a decay chain is. She points in the direction of an expanded periodic table that, despite a year of college chemistry, means about as much to me as a list of shipbuilding supplies from the 1600s. Birnbaum launches into a primer on radiochemistry: Isotopes are chemical elements with too many or too few neutrons in their centers. Some of these are unstable and therefore release energy by shooting out various types of particles. Unstable isotopes are radioactive, and the particles they shoot out are known as ionizing radiation.
As for what a decay chain is: When radioactive isotopes release radiation, they usually turn into another radioactive isotope, which releases radiation until it turns into another radioactive isotope, and so on, until it hits on something stable. The pattern by which a particular isotope morphs is its decay chain. Today, in addition to whatever goes on behind the fence, Los Alamos National Laboratory is the primary producer of certain isotopes whose decay chains make them useful for medical scans, such as PET scans and heart-imaging techniques. Scientists at Los Alamos deliver the parent isotope in a container called a cow. As the parent decays, doctors "milk" the daughter isotope off to image patients' hearts.
Decay chains present both an opportunity and a responsibility for the U.S. government. You can't just throw decaying radioactive isotopes into a landfill, so after the nuclear age and a half-century Cold War with the U.S.S.R., there are caches of radioactive uranium and plutonium isotopes sitting around gradually turning into other stuff. One of these caches is uranium-233, which was originally created for a reactor program and is currently stored at the Oak Ridge National Laboratory in Tennessee. Over the last forty-some years, it has been slowly turning into thorium-229.
Thorium-229's decay chain leads to actinium-225, which is of interest to cancer researchers for several reasons. For one thing, actinium-225's decay chain goes on for several generations. It turns into francium-221, then astatine-217, then bismuth-213, then mostly polonium-213, then lead-209 before finally hitting a hard stop at bismuth-209, which is stable. In most of these generations, the radiation released consists of alpha particles, which can destroy cancer cells but have low tissue penetration—they leave the surrounding healthy cells mostly alone. Currently, all but one of the radioactive isotopes used in cancer treatment release beta radiation, which causes considerably more collateral damage.
If a drug company could attach an atom of actinium-225 to a targeting system—like, say, the kind in CAR-T cells—the actinium-225 could continuously attack cancer for days at a time, like an artificial, radioactive version of the immune system. Newer chemotherapy drugs called antibody-drug conjugates already use this technique, directing chemotherapy agents that are too strong to give intravenously precisely where they are needed. At least two of these, Kadcyla and Adcetris, have already been approved by the FDA (for HER2-positive breast cancer and Hodgkin's lymphoma, respectively).
Los Alamos: Behind leaded glass, robotic arms quality-test isotope production. The goal: Create actinium-225, an isotope that can be used in cancer drugs.
The U.S. system of national laboratories is already in talks with drug companies about making antibody-based radioactive drugs a reality. They seem promising: In a paper released last July in the Journal of Nuclear Medicine, one late-stage prostate cancer patient treated with three cycles of targeted actinium-225 at the University Hospital Heidelberg in Germany went into complete remission and another's tumors disappeared from scans.
But of course, there's a problem: Now that the reactor program and the Cold War are both over, no one is making uranium-233 in the U.S. (or anywhere). And because it takes more than forty years for uranium-233 to turn into enough thorium-229 to be useful, it wouldn't matter much even if they did. There are currently only about fifteen hundred to seventeen hundred millicuries of actinium-225 anywhere in the world, which would just treat one hundred to two hundred patients a year.
Which brings us to the reason Los Alamos has gotten deeply involved in actinium-225 at all: They're going to figure out how to make more from scratch.
Interlude
A roughshod man with bloodshot eyes rolls a cigarette outside a coffee shop in Taos, New Mexico. I can't be sure if he is the backpacker who was playing a flute at this table earlier or a new person. "You a reporter?" he asks.
"Er, yeah. Just got off the phone with a drug company that thinks they can cure cancer."
"A drug for cancer already exists," the man says. "More people need to be looking at marijuana. It can cure all kinds of sicknesses, but the thing is, the government doesn't want people knowing about it."
A light breeze rustles the wind chimes. We are hiding from the sun under a pergola on the shop's back porch. Another man attempts to come to my rescue: "But wasn't Obama trying to change the rules about experimenting—"
"Obama doesn't want to change the rules because he's not like us," says the first man. "He's got pharaoh DNA that they blend with lizard blood up in the mountains." He inclines his chin toward Los Alamos.
"So he's like a monster?" asks the second man.
"Nah, they're physical, like us, but they only have three chakras, so they're not as balanced." He nods, sagely. "Highly carnivorous."
V. Policy Reform: Divided We FallV. Policy Reform: Divided We Fall
Imagine cancer researchers as thousands of ships attempting to cross the Pacific, all with skills and tools that they have perfected in their home countries. Some have expert navigators. Others build the most watertight ships. If someone could combine the skills of the entire group, they could build a supership the likes of which has never been seen. Instead, they seem to communicate mostly by throwing paper airplanes at each other.
Before Biden appointed him, Simon himself was treated for leukemia three years ago at Sloan Kettering.
"All you could do with government-funded academic research, in the age of paper, was share information in person, so you had these huge cancer meetings once a year where everybody holds their research until they get there," says Greg Simon, the executive director of former vice president Joseph Biden's Cancer Moonshot, an initiative launched by the Obama administration in 2016. "We haven't changed it since."
The system of medical journals, subscriptions to which can cost thousands of dollars, are hardly the only baked-in obstacle to progress in cancer research. Clinical trials are still designed the same way they were fifty years ago. Funding, applied for and received in crazed round-robins of grant-writing, tends to reward low-risk experiments. There's secrecy and competition and slowness and inherent bureaucracy. The system wasn't created to be inefficient, but now that it is, it is intractably so.
Just this week, Simon has flown all over the country trying to bring bullheaded institutions with impossibly huge data troves into a single kumbaya circle of progress. This morning, he gave a speech at the 28th Annual Cancer Progress Conference. Now he is entertaining a journalist at a sushi lunch in the lobby of a Manhattan hotel. By rights, he should be asleep at the table with his face on a plate. Instead, he orders plain fish, no rice, in a disarming Southern accent. (Simon is from Arkansas.)
When Simon was twenty-eight, he played drums in a rock band called the Great Zambini Brothers Band. Then he decided to do something with his life, "quit the band, waited tables, went to law school, got a job, and hated it," he says. A friend found him work in Washington and by forty-one, Simon was working in the White House as an aide to then vice president Al Gore. Then he cofounded a Washington think tank called FasterCures. Then he worked as senior vice president for patient engagement at Pfizer. If anyone on earth knows how to get from here to there, Simon is the guy.
"Future." A tricky word for a cancer patient.
Since he left the White House (again) in January, Simon and his team have begun developing, out of a WeWork space, a spin-off of the Cancer Moonshot they're calling the Biden Cancer Initiative. It will be its own separate nonprofit, apart from government or charity. Its goal: Fix policy and make connections so that those with the expertise to cure cancer have a clear path to the finish line.
To achieve such a feat, Simon will work against a scientific version of the tragedy of the commons—an economic theory in which each person, acting in his own best interest, screws up the whole for everyone else. Convincing people and institutions to act against their own best interest will be much like governing, which is to say, slow and impossible. And yet it's hard not to believe in Biden, a man who helped run the most powerful country in the world at the same time he lost his own son to brain cancer.
"We won't be funding research. The world doesn't need another foundation with money," says Simon. "What it needs is someone like Biden, who's willing to knock heads together . . ." He pauses. "Or cajole heads together, to make the changes that everyone has an excuse not to do: I wanna make money, I want tenure, I wanna get published, I want this, I want that."
The fragmentation in medical research—the lone ships out on the ocean—doesn't exist as much in other sciences, says Simon, because scientists in other disciplines have no choice but to share equipment: telescopes or seismology sensors or space shuttles. Industries that have managed to work together have sent humans to the moon. "We don't even know how much progress we could make in our cancer enterprise because we've never had it up and running at a level that would be optimal," he says.
Philadelphia: Greg Simon, who ran Biden\'s Moonshot, at a breakfast conference.
Simon himself had cancer. Three years ago. It was CLL. "I found it through a physical," he says. "I never had any of the raging symptoms, like bleeding. During the chemo I didn't notice it at all. Zero side effects. I thought I'd lose my hair so I grew a beard. But I didn't."
Interlude
"You are writing. Are you writer?" asks the flight attendant on Delta Flight 3866 from LaGuardia to Memphis in a thick Eastern European accent. It's a late flight—post-work—and many of the passengers are asleep. My reading light is one of just three that are illuminated.
"I had cancer," she says. "Breast cancer. I still have no boobs. After my surgery, they put in a balloon that they inflate step by step. After a few weeks I say to the doctor, 'I am still as flat as pancake!' And he says, 'Ah, there must be a hole.' "
The flight is turbulent, so the flight attendant perches on the arm of the seat in front of me. "I go home after surgery and I have a chill, so I take my medication—they give you such powerful medication—and I sleep. Thank god my friend came over and said I had to take a shower, because I took off the bandages and it was as red as this!" She points to the crimson bit of her Delta pin.
The flight attendant, diagnosed with stage 3a breast cancer, had developed a blood infection, and had to go to the hospital for intravenous antibiotics. After that, she had eight rounds of chemotherapy and thirty-three of radiation.
"There was so much pain, but I had to walk through the pain. I made myself," she says. "I wrote 'I love you' on my mirror in lipstick. When you're single and you have cancer and you look at yourself, you need to read that. What else is there to do?"
CAR-T Cells Explained
To make a CAR-T cell, doctors remove some of a patient's T-cells using a process similar to dialysis. In a lab, they use a gene-editing technique, such as infecting the T-cells with a modified virus, to add in a new receptor (left). This new receptor, called a chimeric antigen receptor, or CAR, is like a key that matches a very specific lock on the surface of cancer cells.
When doctors return the mutant T-cells to the patient, they flow through the body, attach to the cancer's lock, and start trying to kill it the same way they would a cell infected by a virus. First, the T-cells release a chemical called perforin, which makes a little hole in the cancer cell. Then the T-cells release cytotoxins, which flow in through the hole until the cancer cell dies.
VI. Silicon Valley: The Brain
Through the floor-to-ceiling windows of the Parker Institute for Cancer Immunotherapyin San Francisco are the windswept headlands of the Golden Gate Bridge, the Pacific Ocean, and a frothy coral rotunda called the Palace of Fine Arts.
"Would you like a water?" asks the center's publicist when I visit. "Still or sparkling?"
Of all the cancer centers I visited, the Parker Institute seemed the most like the future of medicine. The office, a few doors from Lucasfilm, has one of those pristine, snack-filled tech startup kitchens with glass jars and a microwave that pulls out like an oven. On a table in the reception area sits a set of glittery silver pamphlets the size of small yearbooks explaining the mission.
San Francisco: Whiteboarding at Napster founder Sean Parker\'s R&D operation.
The man behind the Parker Institute is serial entrepreneur Sean Parker, the cofounder of Napster and intermittent recipient of richly deserved tabloid jabs. Parker doesn't have the most sterling humanitarian reputation: In the movie The Social Network, Justin Timberlake portrayed him as a narcissistic party boy who screws over one of Facebook's cofounders and is arrested for cocaine possession. Parker was fined $2.5 million by the California Coastal Commission for building the set of his $10 million Lord of the Rings–themed wedding (complete with fake ruins, waterfalls, and a cottage) in an ecologically sensitive area. And yet, a little over a year ago, the same man donated $250 million to fund the study of immunotherapy at a lavish backyard gala featuring performances by John Legend, Lady Gaga, and the Red Hot Chili Peppers.
The public story about Parker's philanthropic effort is that it stemmed from the death of his close friend, film producer Laura Ziskin, to recurrent breast cancer. According to Jeff Bluestone, the Parker Institute's president and CEO (and, incidentally, the researcher who characterized CTLA-4 around the same time as Jim Allison), Parker was interested in cancer long before he met Ziskin. "Sean's been interested in the immune system for much of his life, because he's got asthma, and he's had a serious immunological imbalance," he says, sitting at a polished raw-wood conference table half again as long as a normal conference table. (Parker is extremely allergic to peanuts.) "As long ago as 2004, before Laura got sick again, he thought the immune system was going to be the answer. He deeply understands a lot of the science. We joke, is he a second-year graduate student? A third-year postdoc? Should he just go get a Ph.D.?"
Parker is not the first very wealthy person who has used his money to combat disease. Several people at the Institute took care to explain how they were different from the Howard Hughes Medical Institute, a science-funding organization founded by the reclusive airman in 1953. A more influential predecessor might be Michael Milken, the Wall Street financier who founded a charity dedicated to family medicine with his brother Lowell in 1982 that supported, among other things, the research that led to Gleevec, the precision-medicine drug. Milken's funds also supported Jim Allison during an important time in his pre-checkpoint-inhibitor-therapy research when his National Institutes of Health grant had briefly lapsed. In 2003, Milken cofounded FasterCures with Greg Simon with the goal of increasing the pace of cures to "all serious diseases."
Houston: A melanoma tumor sample is processed for analysis.
Some would argue that technology entrepreneurs are exactly the people who should be constructing the immaculate future of cancer research conceived by people like Joe Biden and Greg Simon. For one thing, tech entrepreneurs have already disrupted everything else. They understand the fast-moving, coin-chasing world of biotech development. Parker himself has already succeeded at convincing hardheaded institutions to work together. While he was an early investor and board member in the music streaming service Spotify, he negotiated with Universal and Warner to convince them to participate.
The Parker Institute's fundamental accomplishment thus far has been to do exactly this in cancer research. From the beginning, six academic research institutions signed on to work together under the Parker Institute's umbrella: Memorial Sloan Kettering; MD Anderson; Penn Medicine; Stanford Medicine; University of California, Los Angeles; and University of California, San Francisco. The six, along with independent investigators at a few other research institutions, agree to share research data and work together on goals and projects without getting hung up on institutional constraints, such as intellectual property. In return, they get two things: money, which every cancer researcher needs; and guidance, which is equally pressing but not necessarily as obvious.
"To become a leader in this field, to be a Carl June or a Jim Allison, you usually have to be a bit—not myopic, but a little blind," says Fred Ramsdell, the Parker Institute's vice president of research. This is common in science. To understand and work on a complicated concept, a researcher has to shut out the noise of everything except his exact area of expertise. Someone who works on checkpoint inhibitor therapies in melanoma, for example, might not see much use in reading about ovarian cancer detectors made out of nanocarbon—until suddenly it's the exact bridge to his own next level of progress.
Microsoft believes A.I. will do a better job of parsing papers for insights than people can.
"If a person knows nothing about nanoparticles, I can step in and say, Hey, this nanoparticle thing might be exactly what you need," says Ramsdell. "I spend a lot of time trying to develop relationships between people who might not always do so on their own." Some of those relationships are between researchers themselves. Others are between M.D.'s and Ph.D.'s, or between researchers and drug companies, or engineering companies, or the U.S. Patent Office. It doesn't really matter, so long as the arrangement furthers knowledge.
Up the coast in Seattle, another tech company is attempting to help cancer researchers cross entrenched divides. Microsoft's Project Hanover has already made considerable progress on creating a combined, searchable repository of the scientific news released every month by cancer researchers all over the world. The idea is that artificial intelligence will do a better job of parsing the vast landscape of scientific papers (those paper airplanes flying between ships) for insights. Rather than fallible humans trying to catch every valuable new detail as papers fly out of scientific clearinghouses, the system will do it for them, considering every possible combination of targeted drugs and genetic interactions in less time and more detail than it would take a team of educated humans.
Microsoft calls this the reasoning bottleneck. In a way, they're tackling it the same way the Parker Institute is. The same way the human body does: They're adding a brain.
Interlude
San Francisco. It's late. At the restaurant, there is a man seated at the chef's table when I arrive, drinking a balloon glass of red wine.
"How's the food?" the man asks after a good half hour. It is delicious—a buttery bucatini with lamb ragu and bread crumbs. The man has lived down the street from this restaurant for years. He's a former tech entrepreneur who is now a project manager for a retail company. I tell him what I am writing.
"That's a hell of a coincidence," the man says. "I just flew home from watching my father die of cancer."
"Jesus, I'm sorry."
"He's still there. With my sister. He told me he was tired of feeling like he was on death watch. He told me I should just go. So I went."
He sips his wine.
VII. Hope
Norwalk, Connecticut: A patient uses the DigniCap at Smilow Family Breast Health Center.
What you see after a person has been debilitated by cancer and lived are the scars. The missing jaw or breast. The colostomy bag. Hair that has grown back curly or coarse or gray in patches. Tattoos that mark the paths of radiation beams. The disease that contains all of human biology leaves no one unchanged. There is before cancer, and then there is after.
Above Patrick Garvey's desk, on the top shelf of a bookcase, sits a stack of brown resin jawbones—dozens of them, mostly the mandible, or bottom jaw, which is commonly replaced with a bit of lower leg bone when it has to be removed because it is shot through with cancer. Every jawbone above Garvey's desk is a relic from a surgery he has performed at MD Anderson over the course of three years—more than two hundred patients whose faces are forever altered by their interaction with the disease.
Later today, Garvey will operate on a man with a more difficult case—a large tumor in the maxilla, or top jaw—as part of two surgical teams. The first team will remove the tumor and most of the bone, including the man's eye, and then Garvey's team will remove a piece of the man's fibula along with its blood supply and use it to reconstruct the man's face. "We'll be here into the night," Garvey says.
This type of surgery is called microvascular reconstruction surgery. It drastically improves life for patients who would otherwise, like late film critic Roger Ebert, no longer be able to eat or talk without support. When it fails, however, it fails impressively: The transferred bone must have the correct blood supply or the body will simply reabsorb it, leaving only the bare metal scaffold the doctor implanted. Human bone is far better suited to the long-term mechanics of chewing and talking than metal is, and a plate without bone to protect it will eventually snap, like a paper clip bent back and forth over and over. Garvey has had to reconstruct jaws that have failed before, leaving patients disfigured and unable to chew properly. For a patient who has already undergone treatment for cancer, the impact of having to have multiple reconstructive face surgeries is harrowing.
To make the surgery simpler, Garvey's team uses 3D-printed cutting guides and robotically milled metal plates to create the most precise reconstruction possible. This is how the brown resin jawbone graveyard above his desk got started. After a patient has a CT scan, a company called Materialise in Plymouth, Michigan, prints the jaw models as well as bolt-on cutting guides that show the surgeons exactly where to saw and reconnect fibula bones to match the person's original bone structure. Another company, in New York, creates a metal scaffold that is meticulously bent so as to re-create the original face angles, so MD Anderson's surgeons don't have to bend an off-the-shelf part into position during the reconstruction.
By all accounts, using 3D-printed guides to reconstruct a human face is an advance at the very edge of cancer medicine, and yet it is still disheartening to look at the statistics. Last year, another 1.7 million Americans were diagnosed with cancer, and almost six hundred thousand died. Since 2004, according to the latest data available, the overall decline in death rates has been just 1.8 percent in men and 1.4 percent in women year over year. The five-year survival rate for pancreatic cancer, which most doctors consider the worst of the worst, sits stubbornly at just 8.2 percent.
Perhaps the cure for cancer seems so elusive because it's a failure of semantics. "Curing cancer" is impossible, and the statistics reflect that: Cancer kills more Americans every two years than those who died in every war we've ever fought. However, helping some cancer patients, the luckiest of the unlucky, live in relative normalcy for years is not just possible. It is happening. The five-year overall cancer survival rate is up from 50 percent in 1975 to 67 percent today. For melanoma, it's 91.7 percent. For prostate cancer, it's 98.6. It will take time for the most promising treatments to trickle down to everyone they might be able to help, but in the meantime, the march continues.
What this has to do with Patrick Garvey is that, even subtly, using 3D-cutting guides to improve plastic surgery shifts the focus of cancer treatment from emergency battlefield triage to matters of aesthetics and psychology that matter months and years down the line. Without saying it, exactly, the field of cancer treatment is acknowledging that cancer could one day become a survivable disease—that even a stage four metastatic cancer patient could survive long enough for normalcy to matter.
There are others on the front lines: At hospitals across the country, women with breast cancer can wear a scalp-cooling system called DigniCap during chemotherapy treatments to reduce the likelihood of hair loss. At MD Anderson, a neuroscientist retrains patients' brains to improve altered nerve sensation caused by chemotherapy. St. Jude hired a psychologist to help teen cancer patients plan to save their eggs or sperm, in case their treatments render them infertile and they want to have a family in the future.
Future. A tricky word for a cancer patient. Who gets to have one is still a function of blind fortune. But all these ideas are starting to come together, and progress is suddenly accelerating. We are at what Crystal Mackall calls "the end of the beginning," and the hope is that one day soon, the miracles will no longer be miracles. They will just be what happens. Until then, we pin our hopes on the incremental or unpredictable improvements—the half measures, the better outcomes. It will always be true that once a person has had that most frightening of conversations with chance, life will be split into two parts—the time before cancer, and the time after it. But for a fortunate few, perhaps the second part can be as good, and even as rich and wonderful and as great as the first.
This article first appeared in the June 2017 issue of Popular Mechanics.
Jacqueline Detwiler-George has a master's degree in neuroscience and has contributed to Wired, Esquire, Fast Company, and Best American Science and Nature Writing. She's the former articles editor at Popular Mechanics and former host of the Most Useful Podcast Ever.
-
Our Complete Quiet Luxury Shopping Guide
30 quality closet classics that always have—and always will—endure.
By Emma Childs Published
-
Kaia Gerber and Cindy Crawford Medal in Mother-Daughter Olympic Matching
The pair touched down in Paris wearing coordinating navy looks.
By Julia Marzovilla Published
-
Prince Harry Reveals Royal Family Rift Was Worsened by Tabloids
"Yeah, that’s certainly a central piece to it."
By Amy Mackelden Published
-
Senator Klobuchar: "Early Detection Saves Lives. It Saved Mine"
Senator and breast cancer survivor Amy Klobuchar is encouraging women not to put off preventative care any longer.
By Senator Amy Klobuchar Published
-
I'm an Egg Donor. Why Was It So Difficult for Me to Tell People That?
Much like abortion, surrogacy, and IVF, becoming an egg donor was a reproductive choice that felt unfit for society’s standards of womanhood.
By Lauryn Chamberlain Published
-
The 20 Best Probiotics to Keep Your Gut in Check
Gut health = wealth.
By Julia Marzovilla Published
-
Simone Biles Is Out of the Team Final at the Tokyo Olympics
She withdrew from the event due to a medical issue, according to USA Gymnastics.
By Rachel Epstein Published
-
The Truth About Thigh Gaps
We're going to need you to stop right there.
By Kenny Thapoung Published
-
3 Women On What It’s Like Living With An “Invisible” Condition
Despite having no outward signs, they can be brutal on the body and the mind. Here’s how each woman deals with having illnesses others often don’t understand.
By Emily Shiffer Published
-
The High Price of Living With Chronic Pain
Three women open up about how their conditions impact their bodies—and their wallets.
By Alice Oglethorpe Published
-
I Used to Imagine Murdering the Men I Dated
Falling in love helped me finally figure out why.
By Jessica Amento Published